1 MRI: Fruit Quality Measurements
Michael J. McCarthy, Young Jin Choi and Siwalak Pathaveerat
Department of Food Science and Technology
University of California, Davis, California USA 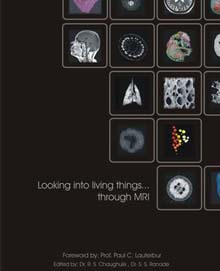
INTRODUCTION
The quality of fruit depends upon both the external and internal
fruit properties. The properties of fruit may vary as a function
of weather, harvest conditions, natural biological diversity
and handling conditions. Fruit purchasers such as retailers
and consumers desire a uniform known external and internal
quality for each fruit. Current state-of-the-art sorting and
sensing technology is primarily focused on external surface
quality features like color. External quality features are
assessed for each and every fruit using automated color sorting
equipment. In contrast, internal quality features are generally
assessed off-line by taking a sampling of fruit for destructive
testing. As the fruit market becomes more competitive and
international there is a greater need to determine internal
quality of fruit to successfully meet market demands and limit
losses. A shipment of citrus fruit that contains a single
fruit infected with a green mold can result in damage to the
entire shipment. This type of damage results in rejection
of the lot and a significant economic loss on the shipment.
Additionally, the fruit producer who sent the mold contaminated
shipment potentially looses the customer to a competing fruit
producer. This type of scenario has increased the interest
in new internal quality sensors that are nondestructive and
can operate at fruit sorting line speeds of 8-12 fruit per
second. Nuclear magnetic resonance imaging (MRI) has been
shown to be an effective method to measure internal fruit
quality (1, 2) and can theoretically operate at the required
speed.
MRI has been demonstrated to be sensitive to a large number
of internal defects and quality factors in fruit including
insect damage, bruising, dry regions, browning and maturity
(1, 3). These quality features and defects are quantified
in MRI using differences in spin-spin relaxation time, diamagnetic
susceptibility, diffusion coefficient, and signal intensity
(4-9). A recent review by Hills and Clark summarizes defects
and quality parameters that have been measured using nuclear
magnetic resonance spectroscopy (1). While MRI has proven
successful at detecting quality attributes and defects additional
knowledge is needed to actually implement an in-line MRI based
sensor. These steps include the design of suitable hardware,
development of sensitivity scales between MRI parameters and
fruit quality attributes, and testing season-to-season as
well as growing location impact on the developed sensitivity
scales. The development of data on seasonal and growing location
impact on MRI parameters, the automated detection of a defect
and the internal spatial variation of fruit properties will
be presented as examples of the next stage in development
of MRI for sorting fruit. These examples will be demonstrated
through detection of freeze damage in Navel oranges, detection
of mold damage in citrus peel and the variation of composition
within an avocado fruit, respectively.
.contd.
Last paragraph of conclusion
of this chapter
..
The distribution of oil and water and hence the spatially
localized maturity of an avocado varies significantly. Achieving
an accurate determination of the average maturity in the fruit
by any measurement technique depends upon the volume of the
sampled flesh. Ideally the entire flesh would be measured.
However for on-line sensors and off-line tests the actual
volume sampled is usually much smaller than the entire fruit.
Consider the case of measuring percent dry matter of an avocado
using the standard microwave drying technique, where only
a small section of the fruit is used for determination of
the percent dry weight. This method uses a thin longitudinal
slice a few mm thick. The result of this procedure could easily
be influenced if the thickness of the slice were not uniform
(e.g. calyx end thicker than stem end). Likewise the NMR technique
determined value can be influenced by what volume is sampled.
Table 8 shows the variation in measured maturity level as
a function of sampled volume size in the chemical shift image.
Each pixel in the image represents volume of [(7.0/32) (7.0/32)
0.2] cm3. As the volume of the measurement is increased the
value of the measured maturity increases towards the average
value for maturity of the avocado. The table demonstrates
that a volume with ~ 2 cm diameter is insufficient to accurately
predict the intensity of the entire slice. The data in Table
8 also explains the results obtained by varying the diameter
of surface coil (results not shown).
References:
1. Hills BP, Clark CK. Quality assessment of horticultural
products by NMR. Annual Reports on NMR Spectroscopy 2003:50:76-120.
2. McCarthy MJ. 1994. Magnetic Resonance Imaging in Foods.
Chapman &Hall. New York, NY.
3. Chen P, McCarthy MJ, Kauten R. NMR for internal quality
evaluation of fruits and vegetables. Trans. ASAE 1989:32:1747-1753.
.contd
till end of 18 references.
(IMAGES:
Out of 9 figures - figure 5 is given below)
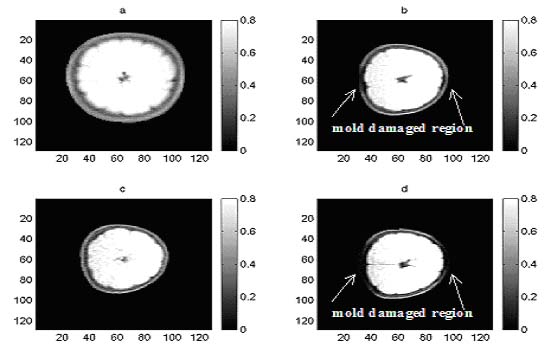
Figure 5. MRI orange images: (a) and (c) for good quality
fruit, and (b) and (d) for mold damaged fruit.
______________________________________________________________________________________________
4 Functional NMR : PLANTS
Markus Rokitta
School of Integrative Biology, The University of Queensland,
St. Lucia, QLD 4072, Australia
and
Universität Würzburg, Experimentelle Physik V
Am Hubland, 97074 Würzburg, Germany
INTRODUCTION
Of all resources a plant needs, water is the most abundant
and at the same time the most limiting one for agricultural
productivity [1]. Therefore, an understanding of the mechanisms
of water uptake and water loss are of particular interest.
First theories about long distance water transport mechanisms
in plants arose with the cohesion theory more than a century
ago [2]. One might expect that all aspects of this problem
have been understood since. However, there is still a very
controversial discussion going on in the very latest literature:
see [3] and reply in the same issue as well as [4,[5,[6].
NMR imaging is particularly suitable for measurement of functional
parameters such as flow due to its non-invasive nature although
the obtainable resolution does not reach the resolution of
optical microscopy. In this context functional means that
physiological parameters can be investigated dynamically over
time. During an experiment one can alter certain conditions
and observe the plant's response to these changes.
ADVANTAGES OF MRI FOR PLANT EXPERIMENTS
The advantages of NMR as compared to other imaging methods
include non-invasive monitoring of anatomy, solute distribution
and time dependent changes of active and passive transport
of substances (water, ions, primary and secondary metabolites)
in plants. No special preparation of the sample is necessary
so that plants can be examined under controlled external conditions
like humidity, temperature, illumination, nutrition and so
on without perturbance by the measurement itself. All parts
of the plant are accessible at any stage of their development.
Advantages of NMR should be obvious when applied to transgenic
plants. The effects of a genetic modification on plant anatomy,
solute transport, metabolism and ways to compensate for genetic
defects can be studied at the quite different level, inaccessible
for other techniques. However, while the spatial resolution
of NMR at the current state of the art is approximately ten
times less compared to optical microscopy, NMR should be seen
as complimentary to other techniques. Meaningful applications
of plant NMR must therefore take advantage of the integrated
observation of intact plants. There are a number of fundamental
questions in plant biology that need to be addressed with
the non-destructive, in vivo, contact-less NMR technique:
How are solutes (water, sugar, lipids, amino acids, ions)
distributed in plants and how is solute distribution related
to structural and functional characteristics of tissues during
development and under stress conditions at the whole plant
or whole organ level?
How does structural and metabolic crosstalk of different organs/tissues
occur within an interactive system, for example within the
seed (seed coat - endosperm - embryo)?
What forces and mechanisms play a role in water and solute
transport in plants?
What effects have genetic modifications on the intact plant?
What compensation mechanisms exist for genetically modified
plants?
.contd.
(IMAGES: Out of 7 figures - figure 5 is given below)
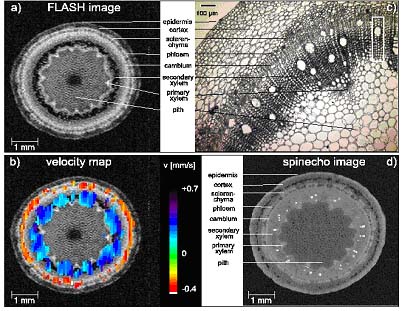
Figure5
Figure 5: Cross-sections of the shoot of a 35 days old intact
and transpiring castor bean plant made by NMR micro-imaging
(a, b, d) and light microscopy (c). (a) FLASH image with a
spatial resolution of 47 m; (b) flow velocity map superimposed
on the previous image; (c) light microscopy; the size of one
pixel in NMR flow imaging (b) is indicated in the upper right
corner of the picture. (d) spin echo image with a spatial
resolution of 12 m. Reproduced from Rokitta et al., Protoplasma,
209:126-131, 1999 [33]
_______________________________________________________________________________________________
19. MR of Lung
David C. Ailion and Gernot Laicher
Department of Physics, University of Utah, 115 South 1400
East, Salt Lake City, Utah 84112
Even though lung is one of the most important organs in the
human body, in the past it has received relatively little
magnetic resonance study. However, with the advent of hyperpolarized
gas NMR imaging, this situation is changing. Proton imaging
has been particularly difficult, primarily for two reasons:
(1) the presence of air-tissue interfaces in the outer (alveolar)
portion of the lung results in severe internal inhomogeneous
broadening of the NMR line which can cause blurring of the
image and (2) the lung is a relatively low density object
which is near much higher density objects (the beating heart
and chest wall) that are moving asynchronously, thereby causing
severe motional artifacts. In this Chapter, techniques are
presented for overcoming both these difficulties in proton
imaging. In particular, asymmetric imaging (as a way to utilize
the inhomogeneous broadening to gain information about lung
microgeometry) and techniques like the rapid line scan and
radial and spiral k-space acquisitions (for reducing or eliminating
motion artifacts) are described. In addition, MRI of hyperpolarized
(hp) gases (3He and 129Xe), which provides an alternate approach
that is much less sensitive to inhomogeneous broadening, is
explained. Applications of NMR to the study of restricted
diffusion of water molecules are presented in which the diffusion
of water within different microscopic compartments can be
distinguished. Finally, applications of these magnetic resonance
techniques to the study of lung diseases like pulmonary edema
and emphysema are discussed.
I. INTRODUCTION
Magnetic resonance imaging (MRI) has made enormous contributions
to medical diagnostics in recent years and has become one
of the major weapons in the physician's arsenal for detecting
and diagnosing diseased regions of the human body. Even though
lung is one of the most essential organs in most animals,
with lung disease being responsible for hundreds of thousands
of human deaths each year, there have been relatively few
applications of MRI to lung, primarily because of technical
difficulties that are peculiar to the lung. These difficulties
arise primarily from three sources: (1) inflated lung has
a much lower water density (approximately 20% that of free
water) than do other biological tissues and will thus be characterized
by a correspondingly smaller proton NMR signal; (2) the NMR
line shape in the outer, mainly parenchymal region (i.e.,
containing the alveoli) is inhomogeneously broadened; and
(3) the NMR image of the lung (a relatively low-density object)
may be affected by the asynchronous motions of nearby high-density
objects (the heart and the chest walls). The inhomogeneous
internal line broadening can cause a blurring of the NMR image
unless special imaging techniques are employed. In Section
II, we discuss the physical origins of this phenomenon and
also describe techniques that will utilize this feature to
allow the imaging of the inflated regions of the lung. The
asynchronous motions of the heart (due to beating) and the
chest wall and lung (due to breathing) give rise to severe
non-local motional artifacts (ghosts) in many MRI applications
(typically those employing the 2D imaging or spin-warp technique).
Several techniques for minimizing these errors will be discussed
in Section III. Section IV describes relaxation time (T1,
T2, and T1?) measurements and techniques as well as possible
mechanisms responsible for these relaxation times. Section
V describes pulse gradient techniques for studying diffusion
with NMR and summarizes the results of measurements of diffusion
of water molecules in lung. These include data acquired using
pulsed magnetic field gradients of moderate strength (~20
gauss/cm) as well as data obtained using ultrahigh static
field gradients (~ 1 Tesla/cm); the results reflect the motion
of water molecules within compartments of different dimensions.
NMR measurements in lung have usually involved proton resonance
and have been limited primarily to NMR in the lung tissue.
Conventional NMR of the airways and gas-exchanging regions
(bronchi, alveoli, ducts) has been very difficult because
of the very low molecular density in the vapor phase. However,
in the last 5-10 years a very promising approach has been
developed for enhancing the NMR signal for certain nuclei
(3He and 129Xe) and has resulted in improvements in the NMR
sensitivity of 4-5 orders of magnitude for these nuclei. The
hyperpolarization (hp) technique, which is described in more
detail in Section VI, involves the transfer of polarization
from laser-polarized electrons of an alkali metal (Rb) to
a noble gas nucleus (3He and 129Xe).
Section VII is a brief summary of some of the medical applications
of the NMR techniques described earlier in this Chapter.
A comprehensive presentation of conventional (i.e., not using
hyperpolarized nuclei) NMR and MRI studies of lung, including
medical applications with emphasis on pulmonary edema, is
given in the book, Application of Magnetic Resonance to the
Study of Lung, edited by A.G. Cutillo [ ]. A brief summary
of lung NMR can be found in an article, "Lung & Mediastinum:
A Discussion of the Relevant NMR Physics", in the Encyclopedia
of Nuclear Magnetic Resonance [ ].
.contd.
There
are 100 references.
______________________________________________________________________________________________
Some images from the book
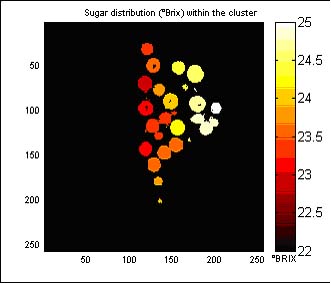
Figure 7 from Ch.2 Wine Grapes - Sugar content is shown as
different intensities of shading.
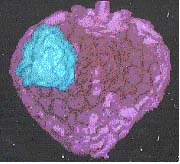 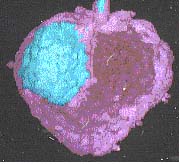
Figure 3 from Chp 6 - Evolution of MRI : Flora To Fauna
Surface rendered images of strawberry fruit infected by Botrytis
cinerea. Image (a) after one day; Image (b) after 2 days.
Fig. 2 from Chp-16MR Spectroscopy in oncology
(A) T1-weighted sagittal contrast enhanced MR image of a patient
suffering from brain stem glioma showing the voxel location
from which the proton MR spectrum is obtained.
(B) Water suppressed proton MR spectrum from an 20 x 20 x
20 mm3 voxel recorded using PRESS sequence that an echo time
of 270 ms with a repetition time of 2 s.
|